28 april 2022: Zie ook dit artikel: https://kanker-actueel.nl/pdt-fotodynamische-therapie-beloftevol-bij-inoperabele-alvleesklierkanker-blijkt-uit-verschillende-studies.html
Zie ook in gerelateerde artikelen
28 april 2022: Bron: Cancers 2021, 13(17), 4354; Received: 30 June 2021 / Revised: 16 August 2021 / Accepted: 26 August 2021 / Published: 28 August 2021
PDT - Foto Dynamische Therapie zou voor alvleesklierkanker een goede behandelingsoptie kunnen zijn, al of niet in combinatie met andere behandelingen, waaronder immuuntherapie. Blijkt uit een grote reviewstudie.
Alvleesklierkanker - Pancreas ductaal adenocarcinoom (PDAC) is een van de moeilijkst te behandelen vormen van kanker. Zeker als het al is uitgezaaid bij de diagnose, wat heel vaak voorkomt. Bovendien ligt de alvleesklier dicht bij het hart en grote slagaders waardoor een operatie al snel niet mogelijk is. Slechts 10%–20% van alle alvleesklierkankertumoren zijn operabel en recidieven treden heel vaak op bij alvleesklierkanker. De mediane 5-jaars overleving voor alvleesklierkanker ligt rond de 10 procent.
Bovendien blijken alvleeskliertumoren veel minder dendritische cellen te hebben waardoor immuuntherapie vaak niet aanslaat bij deze vorm van kanker. (zie dit artikel over dendritische cellen bij o.a. alvleeskliertumoren).
Hoewel dr. Casper van Eyck in 2020 in de publiciteit kwam met een studie met dendritische celtherapie waarbij 9 van de 10 deelnemende alvleesklierkankerpatiënten na anderhalf jaar nog vrij waren van kanker. (zie dit artikel o.a.) Echter de studie is tot op vandaag nog niet gepubliceerd, althans ik kan de studie niet vinden. Dit was REACtiVe-1 het studieprotocol van de studie maar ik zie nog geen publicatie in een Medisch Vakblad van deze studie.
Wel is er een vervolgstudie REACTiVe-2 in het ErasmusMC geopend en daaraan kunnen nog steeds patiënten aan deelnemen, zie dit studieprotocol. In die studie wordt gebruik gemaakt van een CD40 agonis, zoals ook in deze dierstudie is gedaan. Ook weten we dat er in het Erasmus MC muizenstudies lopen met PDT met bremachlorin in combinatie met vormen van immuuntherapie. Met verrassend goede resultaten.
Maar alvleesklierkanker is dus heel moeilijk te behandelen. Ook veel studies met verschillende vormen van chemotherapie, radiotherapie - bestraling, personalised medicin en combinatiebehandelingen hebben over het algemeen tot nu toe geen echte verbetering van de overleving opgeleverd voor patiënten met een inoperabele vorm van alvleesklierkanker.
Fotodynamische therapie (PDT) is een op fotochemie gebaseerde benadering die selectieve celdoding mogelijk maakt met behulp van tumorlokaliserende middelen die worden geactiveerd door zichtbaar of nabij-infrarood licht. PDT - Foto Dynamische Therapie bij alvleesklierkanker biedt verschillende voordelen. Afhankelijk van de lokalisatie van de alvleeskliertumor, kan PDT doelen in tumorcellen direct beschadigen of veranderen. Bovendien, aangezien het zichtbare of nabij-infrarood licht dat in PDT - Foto Dynamische Therapie wordt gebruikt niet-ioniserend is, heeft PDT niet de toenemende toxiciteit die geassocieerd wordt met radiotherapie. [12,23].
In tegenstelling tot ioniserende straling is een groot manco bij PDT dat laserlicht een beperkte penetratie van rode en nabij-infrarode golflengten in weefsel heeft. Lichtafgifte voor interne plaatsen zoals de pancreas vereist een zorgvuldige planning van de behandeling en dosimetrie = berekening van de doses, hoewel er innovatieve oplossingen zijn ontwikkeld en ook bewezen in studies. Zo wordt PDT al langer via licht op een glasvezelkabeltje onder geautomatiseerde tomografische begeleiding gebruikt bij tumoren in het lichaam om de beperking van lichtverzwakking in weefsel te voorkomen.
Een beeld uit het studieverslag hoe PDT ook kan worden gegeven via een glasvezelkabeltje bij alvleeskliertumoren.
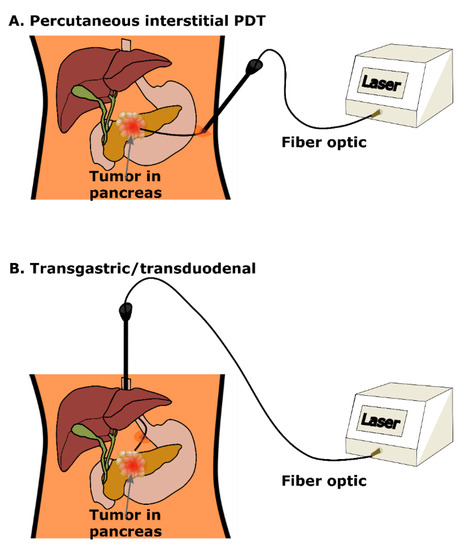
Andrei Reshetnikov vertelde ons dat hij dat al 15 jaar soms toepast in Rusland. We hebben verschillende oncologische chirurgen in Nederland en België afgelopen half jaar aangeschreven of zij deze manier van PDT - Foto Dynamische Therapie samen met bremachlorin wilden toepassen via bv een kijkoperatie bij tumoren in het buikvlies maar zij durven dit niet aan vertelden zij ons. Is toch wel beetje vreemd als je leest dat deze manier van PDT toepassen wel al veel langer en veelvuldig wordt toegepast wereldwijd. Maar goed het is niet anders. Mocht een oncoloog of oncologisch chirurg dit lezen en belangstelling hebben we kunnen haar of hem altijd in contact brengen met Andrei.
In het studierapport staat uitgebreid beschreven met literatuurverwijzingen hoe en wanneer PDT - Foto Dynamische therapie bij alvleesklierkanker kan worden toegepast. Klik op de titel van het abstract.
Photodynamic Therapy for Pancreatic Ductal Adenocarcinoma
1
Department of Physics, University of Massachusetts at Boston, Boston, MA 02125, USA
2
Department of Pathology, BIDMC Cancer Center/Harvard Medical School, Boston, MA 02215, USA
*
Author to whom correspondence should be addressed.
Academic Editors: Sumit Sahni, Anubhav Mittal, Jaswinder Samra and Michael Höpfner
Cancers 2021, 13(17), 4354; https://doi.org/10.3390/cancers13174354
Received: 30 June 2021 / Revised: 16 August 2021 / Accepted: 26 August 2021 / Published: 28 August 2021
(This article belongs to the Special Issue Recent Advances in Pancreatic Ductal Adenocarcinoma)
Simple Summary
Pancreatic ductal adenocarcinoma (PDAC) is among the most lethal of human cancers. Numerous clinical trials evaluating various combinations of chemotherapy and targeted agents and radiotherapy have failed to provide meaningful improvements in survival. A growing number of studies however have indicated that photodynamic therapy (PDT) may be a viable approach for treatment of some pancreatic tumors. PDT, which uses light to activate a photosensitizing agent in target tissue, has seen widespread adoption primarily for dermatological and other applications where superficial light delivery is relatively straightforward. Advances in fiber optic light delivery and dosimetry however have been leveraged to enable PDT even for challenging internal sites, including the pancreas. The aim of this article is to help inform future directions by reviewing relevant literature on the basic science, current clinical status, and potential challenges in the development of PDT as a treatment for PDAC.
Pancreatic ductal adenocarcinoma (PDAC) is among the most lethal of human cancers. Clinical trials of various chemotherapy, radiotherapy, targeted agents and combination strategies have generally failed to provide meaningful improvement in survival for patients with unresectable disease. Photodynamic therapy (PDT) is a photochemistry-based approach that enables selective cell killing using tumor-localizing agents activated by visible or near-infrared light. In recent years, clinical studies have demonstrated the technical feasibility of PDT for patients with locally advanced PDAC while a growing body of preclinical literature has shown that PDT can overcome drug resistance and target problematic and aggressive disease. Emerging evidence also suggests the ability of PDT to target PDAC stroma, which is known to act as both a barrier to drug delivery and a tumor-promoting signaling partner. Here, we review the literature which indicates an emergent role of PDT in clinical management of PDAC, including the potential for combination with other targeted agents and RNA medicine.
7. Emerging and Future Directions
Given the noted role of stroma in PDAC progression and as a barrier to drug delivery, the concept of therapeutically targeting PDAC stroma has emerged as a potentially important strategy. As discussed here, multiple lines of evidence suggest that PDT may have some role to play in this context. In a 3D PDAC co-culture model, it was shown that while the nodules in the presence of fibroblasts were more chemoresistant, PDT response was enhanced in the presence of fibroblasts [78]. Another study on PDAC cocultured with fibroblasts in monolayer showed non significantly higher PDAC cell death in the presence of non-activated fibroblasts [127]. Furthermore, a study demonstrated that PDT not only depleted stromal fibroblasts, but also interrupted crosstalk with stromal signaling partners that gave rise to enhance tumor survival [128]. Combined with additional evidence that PDT can induce breakdown of ECM components [129], these preclinical results collectively indicate a potential role for PDT in depleting cellular and non-cellular PDAC stromal components to enhance subsequent drug delivery. Clinically this scenario could be leveraged by activating the PS at early timepoints following delivery when initial stromal accumulation is highest.
In consideration of how PDT may play a role in the next generation of cancer therapeutics, the opportunity for interactions with RNA medicine emerges as a potentially exciting avenue of investigation. In recent years, RNA medicine has demonstrated exciting potential for a wide variety of diseases, including, specifically, PDAC, through targeting of microRNAs (miRNAs) [130,131,132,133]. MiRNAs are small (~18–25 nucleotides) non-coding RNAs that can bind target mRNAs in a sequence-specific fashion to induce post-transcriptional downregulation. Several studies have already identified miRNAs with significantly altered expression between normal pancreas and PDAC tissues; among them, miR-21, miR-196a, and miR-196b, which are strongly correlated with decreased survival [134,135]. In a mouse model of miR-21 over-expression, it has been revealed that the mice develop tumors in tissue where miR-21 is over-expressed, and that these tumors depend on the continued expression of miR-21 for survival [136]. These lines of evidence highlight the role of ‘oncomiR addiction’ in regulating key pathways promoting tumor growth, survival, and chemoresistance [137]. Furthermore, miR-21 depletion using a nanoparticle to carry an anti-miRNA inhibitor also inhibits organoid growth, suggesting the potential of this approach as a therapeutic strategy [130]. At the same time, this approach opens new potential avenues for synergy with PDT. Inhibition of miR-21 has been shown to increase levels of the pro-apoptotic factor BAX, while PDT with verteporfin is known to target anti-apoptotic factors BCL-2 and BCL-XL [138,139]. Similarly, targeting of another PDAC onco-miR, miR-196b, has also been shown to promote resistance to late-stage apoptosis in PDAC cells [140]. Combination of PDT with selective therapeutic inhibition of these oncomiRs could synergistically increase the Bax/Bcl-2 ratio (pro-/anti-apoptotic) in PDAC cells and tip the balance toward apoptosis in these otherwise stubbornly drug-resistant cells. In addition to synergizing at the molecular level, combination with PDT could also enhance delivery of RNA medicines through depletion of stromal components. As noted above, the notoriously dense fibrotic stroma in PDAC is problematic for delivery of virtually all therapeutic agents, and this may be especially true for RNA medicine. While various anti-miRNA strategies have been discussed for the past decade, a lack of adequate delivery to most disease tissues has restricted current therapeutic uses to liver and kidney disease. Collectively, these observations point to the potential benefits of leveraging nanoparticle delivery systems that could simultaneous carry light-activated agents for PDT to target PDAC stroma for enhanced delivery of RNA medicine agents, while at the same time priming tumor cells for enhanced biological response to these therapies.
8. Conclusions
Collectively, the literature points to multiple significant roles for PDT in the clinical management of PDAC. The clinical studies discussed above have established the techniques for light delivery which enable PDT as a primary treatment for locally advanced PDAC, which could play a key role especially for disease which is unresectable. However, biological responses to PDT may also synergize with systemic therapies as part of a complete treatment strategy. At the same time, active and ongoing research continues to reveal new roles for PDT and its potential to interact with other promising strategies that are just beginning to emerge.
Author Contributions
Conceptualization, V.K., F.J.S. and J.P.C.; methodology, V.K. and J.C.; software, V.K.; validation, V.K., F.J.S. and J.P.C.; formal analysis, V.K. and J.P.C.; investigation, V.K. and J.P.C; resources, J.P.C.; data curation, V.K. and J.P.C.; writing—original draft preparation, V.K.; writing—review and editing, V.K. and J.P.C.; visualization, V.K.; supervision, J.P.C.; project administration, J.P.C.; funding acquisition, V.K., F.J.S. and J.P.C. All authors have read and agreed to the published version of the manuscript.
Funding
We gratefully acknowledge funding from the National Cancer Institute to the UMass Boston-Dana Farber/Harvard Cancer Center U54 Partnership (U54CA156734). We thank Oracle and the College of Science and Mathematics at UMass Boston for fellowship support to VK.
Conflicts of Interest
The authors declare no conflict of interest.
References
- Mizrahi, J.D.; Surana, R.; Valle, J.W.; Shroff, R.T. Pancreatic cancer. Lancet 2020, 395, 2008–2020. [Google Scholar] [CrossRef]
- Ryan, D.P.; Hong, T.S.; Bardeesy, N. Pancreatic adenocarcinoma. N. Engl. J. Med. 2014, 371, 1039–1049. [Google Scholar] [CrossRef]
- Quilbe, A.; Moralès, O.; Baydoun, M.; Kumar, A.; Mustapha, R.; Murakami, T.; Leroux, B.; De Schutter, C.; Thecua, E.; Ziane, L.; et al. An Efficient Photodynamic Therapy Treatment for Human Pancreatic Adenocarcinoma. J. Clin. Med. 2020, 9, 192. [Google Scholar] [CrossRef]
- Castillo, C.F.-D.; Morales-Oyarvide, V.; McGrath, D.; Wargo, J.A.; Ferrone, C.R.; Thayer, S.P.; Lillemoe, K.D.; Warshaw, A.L. Evolution of the Whipple procedure at the Massachusetts General Hospital. Surgery 2012, 152, S56–S63. [Google Scholar] [CrossRef] [PubMed]
- Gagner, M.; Palermo, M. Laparoscopic Whipple procedure: Review of the literature. J. Hepato-Biliary-Pancreatic Surg. 2009, 16, 726–730. [Google Scholar] [CrossRef] [PubMed]
- Adamska, A.; Domenichini, A.; Falasca, M. Pancreatic Ductal Adenocarcinoma: Current and Evolving Therapies. Int. J. Mol. Sci. 2017, 18, 1338. [Google Scholar] [CrossRef] [PubMed]
- Kamisawa, T.; Wood, L.D.; Itoi, T.; Takaori, K. Pancreatic cancer. Lancet 2016, 388, 73–85. [Google Scholar] [CrossRef]
- Burris, H.; Storniolo, A. Assessing clinical benefit in the treatment of pancreas cancer: Gemcitabine compared to 5-fluorouracil. Eur. J. Cancer 1997, 33, S18–S22. [Google Scholar] [CrossRef]
- Conroy, T.; Desseigne, F.; Ychou, M.; Bouché, O.; Guimbaud, R.; Bécouarn, Y.; Adenis, A.; Raoul, J.-L.; Gourgou-Bourgade, S.; De La Fouchardière, C.; et al. FOLFIRINOX versus Gemcitabine for Metastatic Pancreatic Cancer. N. Engl. J. Med. 2011, 364, 1817–1825. [Google Scholar] [CrossRef]
- Conroy, T.; Hammel, P.; Hebbar, M.; Ben Abdelghani, M.; Wei, A.C.; Raoul, J.-L.; Choné, L.; Francois, E.; Artru, P.; Biagi, J.J.; et al. FOLFIRINOX or Gemcitabine as Adjuvant Therapy for Pancreatic Cancer. N. Engl. J. Med. 2018, 379, 2395–2406. [Google Scholar] [CrossRef]
- Agostinis, P.; Berg, K.; Cengel, K.A.; Foster, T.H.; Girotti, A.W.; Gollnick, S.O.; Hahn, S.M.; Hamblin, M.R.; Juzeniene, A.; Kessel, D.; et al. Photodynamic therapy of cancer: An update. CA Cancer J. Clin. 2011, 61, 250–281. [Google Scholar] [CrossRef]
- Bown, S.G.; Rogowska, A.Z.; EWhitelaw, D.; Lees, W.R.; Lovat, L.; Ripley, P.; Jones, L.; Wyld, P.; Gillams, A.; Hatfield, A.W.R. Photodynamic therapy for cancer of the pancreas. Gut 2002, 50, 549–557. [Google Scholar] [CrossRef]
- Hanada, Y.; Pereira, S.P.; Pogue, B.; Maytin, E.V.; Hasan, T.; Linn, B.; Mangels-Dick, T.; Wang, K.K. EUS-guided verteporfin photodynamic therapy for pancreatic cancer. Gastrointest. Endosc. 2021, 94, 179–186. [Google Scholar] [CrossRef]
- Mahmoudi, K.; Garvey, K.L.; Bouras, A.; Cramer, G.; Stepp, H.; Raj, J.G.J.; Bozec, D.; Busch, T.M.; Hadjipanayis, C.G. 5-aminolevulinic acid photodynamic therapy for the treatment of high-grade gliomas. J. Neuro-Oncol. 2019, 141, 595–607. [Google Scholar] [CrossRef]
- Schmidt-Erfurth, U.; Hasan, T. Mechanisms of Action of Photodynamic Therapy with Verteporfin for the Treatment of Age-Related Macular Degeneration. Surv. Ophthalmol. 2000, 45, 195–214. [Google Scholar] [CrossRef]
- O’Connor, A.E.; Gallagher, W.; Byrne, A.T. Porphyrin and Nonporphyrin Photosensitizers in Oncology: Preclinical and Clinical Advances in Photodynamic Therapy. Photochem. Photobiol. 2009, 85, 1053–1074. [Google Scholar] [CrossRef] [PubMed]
- Alsaab, H.O.; Alghamdi, M.S.; Alotaibi, A.S.; Alzhrani, R.; Alwuthaynani, F.; Althobaiti, Y.; Almalki, A.H.; Sau, S.; Iyer, A.K. Progress in Clinical Trials of Photodynamic Therapy for Solid Tumors and the Role of Nanomedicine. Cancers 2020, 12, 2793. [Google Scholar] [CrossRef]
- Queirós, C.; Garrido, P.M.; Silva, J.M.; Filipe, P. Photodynamic therapy in dermatology: Beyond current indications. Dermatol. Ther. 2020, 33, 13997. [Google Scholar] [CrossRef] [PubMed]
- Simone, C.B.; Cengel, K.A. Photodynamic Therapy for Lung Cancer and Malignant Pleural Mesothelioma. Semin. Oncol. 2014, 41, 820–830. [Google Scholar] [CrossRef]
- Celli, J.P.; Spring, B.Q.; Rizvi, I.; Evans, C.L.; Samkoe, K.S.; Verma, S.; Pogue, B.; Hasan, T. Imaging and Photodynamic Therapy: Mechanisms, Monitoring, and Optimization. Chem. Rev. 2010, 110, 2795–2838. [Google Scholar] [CrossRef]
- Baptista, M.S.; Cadet, J.; Di Mascio, P.; Ghogare, A.A.; Greer, A.; Hamblin, M.R.; Lorente, C.; Nunez, S.C.; Ribeiro, M.S.; Thomas, A.H.; et al. Type I and Type II Photosensitized Oxidation Reactions: Guidelines and Mechanistic Pathways. Photochem. Photobiol. 2017, 93, 912–919. [Google Scholar] [CrossRef]
- Sun, J.; Du, K.; Diao, J.; Cai, X.; Feng, F.; Wang, S. GSH and H2O2 Co-Activatable Mitochondria-Targeted Photodynamic Therapy under Normoxia and Hypoxia. Angew. Chem. Int. Ed. 2020, 59, 12122–12128. [Google Scholar] [CrossRef]
- Sorrin, A.J.; Ruhi, M.K.; Ferlic, N.A.; Karimnia, V.; Polacheck, W.J.; Celli, J.P.; Huang, H.; Rizvi, I. Photodynamic Therapy and the Biophysics of the Tumor Microenvironment. Photochem. Photobiol. 2020, 96, 232–259. [Google Scholar] [CrossRef] [PubMed]
- Mikvy, P.; Messman, H.; MacRobert, A.J.; Pauer, M.; Sams, V.R.; Davies, C.L.; Stewart, J.C.; Bown, S.G. Photodynamic therapy of a transplanted pancreatic cancer model using meta-tetrahydroxyphenylchlorin (mTHPC). Br. J. Cancer 1997, 76, 713–718. [Google Scholar] [CrossRef] [PubMed]
- Schroder, T.; Chen, I.W.; Sperling, M.; Bell, R.H., Jr.; Brackett, K.; Joffe, S.N. Hematoporphyrin derivative uptake and photodynamic therapy in pancreatic carcinoma. J. Surg. Oncol. 1988, 38, 4–9. [Google Scholar] [CrossRef] [PubMed]
- Nuutinen, P.; Chatlani, P.; Bedwell, J.; MacRobert, A.; Phillips, D.; Bown, S. Distribution and photodynamic effect of disulphonated aluminium phthalocyanine in the pancreas and adjacent tissues in the Syrian golden hamster. Br. J. Cancer 1991, 64, 1108–1115. [Google Scholar] [CrossRef]
- Evrard, S.; Keller, P.; Hajri, A.; Balboni, G.; Mendoza-Burgos, L.; Damgé, C.; Marescaux, J.; Aprahamian, M. Experimental pancreatic cancer in the rat treated by photodynamic therapy. BJS 2005, 81, 1185–1189. [Google Scholar] [CrossRef]
- Huggett, M.T.; Jermyn, M.; Gillams, A.; Illing, R.; Mosse, S.; Novelli, M.; Kent, E.; Bown, S.G.; Hasan, T.; Pogue, B.W.; et al. Phase I/II study of verteporfin photodynamic therapy in locally advanced pancreatic cancer. Br. J. Cancer 2014, 110, 1698–1704. [Google Scholar] [CrossRef] [PubMed]
- Pogue, B.W.; Elliott, J.T.; Kanick, S.C.; Davis, S.C.; Samkoe, K.S.; Maytin, E.V.; Pereira, S.P.; Hasan, T. Revisiting photodynamic therapy dosimetry: Reductionist & surrogate approaches to facilitate clinical success. Phys. Med. Biol. 2016, 61, R57–R89. [Google Scholar] [CrossRef] [PubMed]
- Wilson, B.C.; Patterson, M.S.; Lilge, L. Implicit and explicit dosimetry in photodynamic therapy: A New paradigm. Lasers Med. Sci. 1997, 12, 182–199. [Google Scholar] [CrossRef]
- Kim, M.M.; Darafsheh, A. Light Sources and Dosimetry Techniques for Photodynamic Therapy. Photochem. Photobiol. 2020, 96, 280–294. [Google Scholar] [CrossRef] [PubMed]
- Chan, H.-H.; Nishioka, N.S.; Mino, M.; Lauwers, G.Y.; Puricelli, W.P.; Collier, K.N.; Brugge, W.R. EUS-guided photodynamic therapy of the pancreas: A pilot study. Gastrointest. Endosc. 2004, 59, 95–99. [Google Scholar] [CrossRef]
- Choi, J.-H.; Oh, D.; Lee, J.H.; Park, J.-H.; Kim, K.-P.; Lee, S.S.; Lee, Y.-J.; Lim, Y.-S.; Song, T.J.; Lee, S.S.; et al. Initial human experience of endoscopic ultrasound-guided photodynamic therapy with a novel photosensitizer and a flexible laser-light catheter. Endosc. 2015, 47, 1035–1038. [Google Scholar] [CrossRef] [PubMed]
- DeWitt, J.M.; Sandrasegaran, K.; O’Neil, B.; House, M.G.; Zyromski, N.J.; Sehdev, A.; Perkins, S.M.; Flynn, J.; McCranor, L.; Shahda, S. Phase 1 study of EUS-guided photodynamic therapy for locally advanced pancreatic cancer. Gastrointest. Endosc. 2019, 89, 390–398. [Google Scholar] [CrossRef] [PubMed]
- Kota, J.; Hancock, J.; Kwon, J.; Korc, M. Pancreatic cancer: Stroma and its current and emerging targeted therapies. Cancer Lett. 2017, 391, 38–49. [Google Scholar] [CrossRef]
- Padera, T.P.; Stoll, B.R.; Tooredman, J.B.; Capen, D.; Di Tomaso, E.; Jain, R.K. Cancer cells compress intratumour vessels. Nature 2004, 427, 695. [Google Scholar] [CrossRef]
- Chauhan, V.P.; Martin, J.D.; Liu, H.; Lacorre, D.A.; Jain, S.R.; Kozin, S.V.; Stylianopoulos, T.; Mousa, A.S.; Han, X.; Adstamongkonkul, P.; et al. Angiotensin inhibition enhances drug delivery and potentiates chemotherapy by decompressing tumour blood vessels. Nat. Commun. 2013, 4, 2516. [Google Scholar] [CrossRef]
- Nia, H.; Liu, H.; Seano, G.; Datta, M.; Jones, D.; Rahbari, N.; Incio, J.; Chauhan, V.; Jung, K.; Martin, J.D.; et al. Solid stress and elastic energy as measures of tumour mechanopathology. Nat. Biomed. Eng. 2017, 1, 1–11. [Google Scholar] [CrossRef]
- Stylianopoulos, T.; Martin, J.; Chauhan, V.; Jain, S.R.; Diop-Frimpong, B.; Bardeesy, N.; Smith, B.L.; Ferrone, C.R.; Hornicek, F.J.; Boucher, Y.; et al. Causes, consequences, and remedies for growth-induced solid stress in murine and human tumors. Proc. Natl. Acad. Sci. USA 2012, 109, 15101–15108. [Google Scholar] [CrossRef]
- Nia, H.; Datta, M.; Seano, G.; Huang, P.; Munn, L.L.; Jain, R.K. Quantifying solid stress and elastic energy from excised or in situ tumors. Nat. Protoc. 2018, 13, 1091–1105. [Google Scholar] [CrossRef]
- Nieskoski, M.D.; Marra, K.; Gunn, J.R.; Hoopes, P.J.; Doyley, M.M.; Hasan, T.; Trembly, B.S.; Pogue, B.W. Collagen Complexity Spatially Defines Microregions of Total Tissue Pressure in Pancreatic Cancer. Sci. Rep. 2017, 7, 10093. [Google Scholar] [CrossRef]
- Muz, B.; de la Puente, P.; Azab, F.; Azab, A.K. The role of hypoxia in cancer progression, angiogenesis, metastasis, and resistance to therapy. Hypoxia 2015, 3, 83–92. [Google Scholar] [CrossRef]
- Neesse, A.; Michl, P.; Frese, K.K.; Feig, C.; Cook, N.; Jacobetz, M.A.; Lolkema, M.P.; Buchholz, M.; Olive, K.P.; Gress, T.M.; et al. Stromal biology and therapy in pancreatic cancer. Gut 2011, 60, 861–868. [Google Scholar] [CrossRef]
- Nagy, J.A.; Chang, S.-H.; Shih, S.-C.; Dvorak, A.M.; Dvorak, H.F. Heterogeneity of the Tumor Vasculature. Semin. Thromb. Hemost. 2010, 36, 321–331. [Google Scholar] [CrossRef] [PubMed]
- Guillaumond, F.; Iovanna, J.L.; Vasseur, S. Pancreatic tumor cell metabolism: Focus on glycolysis and its connected metabolic pathways. Arch. Biochem. Biophys. 2014, 545, 69–73. [Google Scholar] [CrossRef]
- Bailey, K.M.; Cornnell, H.H.; Ibrahim-Hashim, A.; Wojtkowiak, J.W.; Hart, C.P.; Zhang, X.; Leos, R.; Martinez, G.V.; Baker, A.F.; Gillies, R.J. Evaluation of the “Steal” Phenomenon on the Efficacy of Hypoxia Activated Prodrug TH-302 in Pancreatic Cancer. PLoS ONE 2014, 9, e113586. [Google Scholar] [CrossRef]
- Tangutoori, S.; Spring, B.Q.; Mai, Z.; Palanisami, A.; Mensah, L.B.; Hasan, T. Simultaneous delivery of cytotoxic and biologic therapeutics using nanophotoactivatable liposomes enhances treatment efficacy in a mouse model of pancreatic cancer. Nanomed. Nanotechnol. Biol. Med. 2016, 12, 223–234. [Google Scholar] [CrossRef] [PubMed]
- Wang, H.W.; Putt, M.E.; Emanuele, M.J.; Shin, D.B.; Glatstein, E.; Yodh, A.G.; Busch, T.M. Treatment-induced changes in tumor oxygenation predict photodynamic therapy outcome. Cancer Res. 2004, 64, 7553–7561. [Google Scholar] [CrossRef] [PubMed]
- McEwan, C.; Owen, J.; Stride, E.; Fowley, C.; Nesbitt, H.; Cochrane, D.; Coussios, C.; Borden, M.; Nomikou, N.; McHale, A.P.; et al. Oxygen carrying microbubbles for enhanced sonodynamic therapy of hypoxic tumours. J. Control. Release 2015, 203, 51–56. [Google Scholar] [CrossRef]
- McEwan, C.; Kamila, S.; Owen, J.; Nesbitt, H.; Callan, B.; Borden, M.; Nomikou, N.; Hamoudi, R.; Taylor, M.A.; Stride, E.; et al. Combined sonodynamic and antimetabolite therapy for the improved treatment of pancreatic cancer using oxygen loaded microbubbles as a delivery vehicle. Biomaterials 2016, 80, 20–32. [Google Scholar] [CrossRef]
- Jahanban-Esfahlan, R.; De La Guardia, M.; Ahmadi, D.; Yousefi, B. Modulating tumor hypoxia by nanomedicine for effective cancer therapy. J. Cell. Physiol. 2018, 233, 2019–2031. [Google Scholar] [CrossRef]
- Zhang, T.; Jiang, Z.; Chen, L.; Pan, C.; Sun, S.; Liu, C.; Li, Z.; Ren, W.; Wu, A.; Huang, P. PCN-Fe(III)-PTX nanoparticles for MRI guided high efficiency chemo-photodynamic therapy in pancreatic cancer through alleviating tumor hypoxia. Nano Res. 2020, 13, 273–281. [Google Scholar] [CrossRef]
- Kang, S.; Gil, Y.-G.; Min, D.-H.; Jang, H. Nonrecurring Circuit Nanozymatic Enhancement of Hypoxic Pancreatic Cancer Phototherapy Using Speckled Ru–Te Hollow Nanorods. ACS Nano 2020, 14, 4383–4394. [Google Scholar] [CrossRef] [PubMed]
- Deng, Y.; Jia, F.; Chen, S.; Shen, Z.; Jin, Q.; Fu, G.; Ji, J. Nitric oxide as an all-rounder for enhanced photodynamic therapy: Hypoxia relief, glutathione depletion and reactive nitrogen species generation. Biomaterials 2018, 187, 55–65. [Google Scholar] [CrossRef] [PubMed]
- Jiang, B.; Zhou, L.; Lu, J.; Wang, Y.; Liu, C.; You, L.; Guo, J. Stroma-Targeting Therapy in Pancreatic Cancer: One Coin With Two Sides? Front. Oncol. 2020, 10, 576399. [Google Scholar] [CrossRef] [PubMed]
- Li, L.; Yang, Z.; Fan, W.; He, L.; Cui, C.; Zou, J.; Tang, W.; Jacobson, O.; Wang, Z.; Niu, G.; et al. In Situ Polymerized Hollow Mesoporous Organosilica Biocatalysis Nanoreactor for Enhancing ROS-Mediated Anticancer Therapy. Adv. Funct. Mater. 2020, 30, 1907716. [Google Scholar] [CrossRef]
- Aguilera, K.; Huang, H.; Du, W.; Hagopian, M.M.; Wang, Z.; Hinz, S.; Hwang, T.H.; Fleming, J.B.; Castrillon, D.H.; Ren, X.; et al. Inhibition of Discoidin Domain Receptor 1 Reduces Collagen-mediated Tumorigenicity in Pancreatic Ductal Adenocarcinoma. Mol. Cancer Ther. 2017, 16, 2473–2485. [Google Scholar] [CrossRef]
- Bailey, J.M.; Swanson, B.J.; Hamada, T.; Eggers, J.P.; Singh, P.K.; Caffery, T.; Ouellette, M.M.; Hollingsworth, M.A. Sonic Hedgehog Promotes Desmoplasia in Pancreatic Cancer. Clin. Cancer Res. 2008, 14, 5995–6004. [Google Scholar] [CrossRef]
- Olive, K.P.; Jacobetz, M.A.; Davidson, C.J.; Gopinathan, A.; McIntyre, D.; Honess, D.; Madhu, B.; Goldgraben, M.A.; Caldwell, M.E.; Allard, D.; et al. Inhibition of Hedgehog Signaling Enhances Delivery of Chemotherapy in a Mouse Model of Pancreatic Cancer. Science 2009, 324, 1457–1461. [Google Scholar] [CrossRef]
- Jimeno, A.; Weiss, G.J.; Miller, W.H.; Gettinger, S.; Eigl, B.; Chang, A.L.S.; Dunbar, J.; Devens, S.; Faia, K.; Skliris, G.; et al. Phase I Study of the Hedgehog Pathway Inhibitor IPI-926 in Adult Patients with Solid Tumors. Clin. Cancer Res. 2013, 19, 2766–2774. [Google Scholar] [CrossRef]
- Amakye, D.; Jagani, Z.; Dorsch, M. Unraveling the therapeutic potential of the Hedgehog pathway in cancer. Nat. Med. 2013, 19, 1410–1422. [Google Scholar] [CrossRef] [PubMed]
- Lee, J.J.; Perera, R.M.; Wang, H.; Wu, D.-C.; Liu, X.S.; Han, S.; Fitamant, J.; Jones, P.D.; Ghanta, K.; Kawano, S.; et al. Stromal response to Hedgehog signaling restrains pancreatic cancer progression. Proc. Natl. Acad. Sci. USA 2014, 111, E3091–E3100. [Google Scholar] [CrossRef] [PubMed]
- Dijke, P.T.; Arthur, H.M. Extracellular control of TGFβ signalling in vascular development and disease. Nat. Rev. Mol. Cell Biol. 2007, 8, 857–869. [Google Scholar] [CrossRef]
- Lin, S.-Z.; Xu, J.-B.; Ji, X.; Chen, H.; Xu, H.-T.; Hu, P.; Chen, L.; Guo, J.-Q.; Chen, M.-Y.; Lu, D.; et al. Emodin inhibits angiogenesis in pancreatic cancer by regulating the transforming growth factor-β/drosophila mothers against decapentaplegic pathway and angiogenesis-associated microRNAs. Mol. Med. Rep. 2015, 12, 5865–5871. [Google Scholar] [CrossRef]
- Chambre, L.; Saw, W.S.; Ekineker, G.; Kiew, L.V.; Chong, W.Y.; Lee, H.B.; Chung, L.Y.; Bretonnière, Y.; Dumoulin, F.; Sanyal, A. Surfactant-Free Direct Access to Porphyrin-Cross-Linked Nanogels for Photodynamic and Photothermal Therapy. Bioconjugate Chem. 2018, 29, 4149–4159. [Google Scholar] [CrossRef]
- Lim, C.-K.; Heo, J.; Shin, S.; Jeong, K.; Seo, Y.H.; Jang, W.-D.; Park, C.R.; Park, S.Y.; Kim, S.; Kwon, I.C. Nanophotosensitizers toward advanced photodynamic therapy of Cancer. Cancer Lett. 2013, 334, 176–187. [Google Scholar] [CrossRef]
- Yu, Z.; Li, H.; Zhang, L.-M.; Zhu, Z.; Yang, L. Enhancement of phototoxicity against human pancreatic cancer cells with photosensitizer-encapsulated amphiphilic sodium alginate derivative nanoparticles. Int. J. Pharm. 2014, 473, 501–509. [Google Scholar] [CrossRef]
- Roh, Y.J.; Kim, J.H.; Kim, I.-W.; Na, K.; Park, J.M.; Choi, M.-G. Photodynamic Therapy Using Photosensitizer-Encapsulated Polymeric Nanoparticle to Overcome ATP-Binding Cassette Transporter Subfamily G2 Function in Pancreatic Cancer. Mol. Cancer Ther. 2017, 16, 1487–1496. [Google Scholar] [CrossRef] [PubMed]
- Li, H.; Yu, Z.; Wang, S.; Long, X.; Zhang, L.-M.; Zhu, Z.; Yang, L. Photosensitizer-encapsulated amphiphilic chitosan derivative micelles: Photoactivity and enhancement of phototoxicity against human pancreatic cancer cells. J. Photochem. Photobiol. B: Biol. 2015, 142, 212–219. [Google Scholar] [CrossRef]
- Jung, H.-Y.; Fattet, L.; Yang, J. Molecular Pathways: Linking Tumor Microenvironment to Epithelial–Mesenchymal Transition in Metastasis. Clin. Cancer Res. 2015, 21, 962–968. [Google Scholar] [CrossRef]
- Polireddy, K.; Chen, Q. Cancer of the Pancreas: Molecular Pathways and Current Advancement in Treatment. J. Cancer 2016, 7, 1497–1514. [Google Scholar] [CrossRef]
- Mahadevan, D.; Von Hoff, D.D. Tumor-stroma interactions in pancreatic ductal adenocarcinoma. Mol. Cancer Ther. 2007, 6, 1186–1197. [Google Scholar] [CrossRef]
- Vonlaufen, A.; Phillips, P.; Xu, Z.; Goldstein, D.; Pirola, R.C.; Wilson, J.; Apte, M. Pancreatic Stellate Cells and Pancreatic Cancer Cells: An Unholy Alliance: Figure 1. Cancer Res. 2008, 68, 7707–7710. [Google Scholar] [CrossRef] [PubMed]
- Kikuta, K.; Masamune, A.; Watanabe, T.; Ariga, H.; Itoh, H.; Hamada, S.; Satoh, K.; Egawa, S.; Unno, M.; Shimosegawa, T. Pancreatic stellate cells promote epithelial-mesenchymal transition in pancreatic cancer cells. Biochem. Biophys. Res. Commun. 2010, 403, 380–384. [Google Scholar] [CrossRef]
- Bachem, M.G.; Schünemann, M.; Ramadani, M.; Siech, M.; Beger, H.; Buck, A.; Zhou, S.; Schmid-Kotsas, A.; Adler, G. Pancreatic carcinoma cells induce fibrosis by stimulating proliferation and matrix synthesis of stellate cells. Gastroenterology 2005, 128, 907–921. [Google Scholar] [CrossRef] [PubMed]
- Öhlund, D.; Handly-Santana, A.; Biffi, G.; Elyada, E.; Almeida, A.S.; Ponz-Sarvise, M.; Corbo, V.; Oni, T.E.; Hearn, S.A.; Lee, E.J.; et al. Distinct populations of inflammatory fibroblasts and myofibroblasts in pancreatic cancer. J. Exp. Med. 2017, 214, 579–596. [Google Scholar] [CrossRef] [PubMed]
- Elyada, E.; Bolisetty, M.; Laise, P.; Flynn, W.F.; Courtois, E.T.; Burkhart, R.A.; Teinor, J.A.; Belleau, P.; Biffi, G.; Lucito, M.S.; et al. Cross-Species Single-Cell Analysis of Pancreatic Ductal Adenocarcinoma Reveals Antigen-Presenting Cancer-Associated Fibroblasts. Cancer Discov. 2019, 9, 1102–1123. [Google Scholar] [CrossRef]
- Karimnia, V.; Rizvi, I.; Slack, F.J.; Celli, J.P. Photodestruction of Stromal Fibroblasts Enhances Tumor Response to PDT in 3D Pancreatic Cancer Coculture Models. Photochem. Photobiol. 2021, 97, 416–426. [Google Scholar] [CrossRef]
- Egeblad, M.; Rasch, M.G.; Weaver, V.M. Dynamic interplay between the collagen scaffold and tumor evolution. Curr. Opin. Cell Biol. 2010, 22, 697–706. [Google Scholar] [CrossRef] [PubMed]
- Reader, C.S.; Vallath, S.; Steele, C.W.; Haider, S.; Brentnall, A.; Desai, A.; Moore, K.M.; Jamieson, N.; Chang, D.; Bailey, P.; et al. The integrin αvβ6 drives pancreatic cancer through diverse mechanisms and represents an effective target for therapy. J. Pathol. 2019, 249, 332–342. [Google Scholar] [CrossRef]
- Turaga, R.C.; Sharma, M.; Mishra, F.; Krasinskas, A.; Yuan, Y.; Yang, J.J.; Wang, S.; Liu, C.; Li, S.; Liu, Z.-R. Modulation of Cancer-Associated Fibrotic Stroma by An Integrin αvβ3 Targeting Protein for Pancreatic Cancer Treatment. Cell. Mol. Gastroenterol. Hepatol. 2021, 11, 161–179. [Google Scholar] [CrossRef]
- Jin, S.; Lee, W.-C.; Aust, D.; Pilarsky, C.; Cordes, N. β8 Integrin Mediates Pancreatic Cancer Cell Radiochemoresistance. Mol. Cancer Res. 2019, 17, 2126–2138. [Google Scholar] [CrossRef] [PubMed]
- Mei, Y.; Li, Y.; Yang, X.; Zhou, C.; Li, Z.; Zheng, X.; Ye, J.; Li, C.; Zhang, X.; Yuan, J.; et al. An optimized integrin α6-targeted peptide for positron emission tomography/magnetic resonance imaging of pancreatic cancer and its precancerous lesion. Clin. Transl. Med. 2020, 10, e157. [Google Scholar] [CrossRef]
- Hamidi, H.; Ivaska, J. Every step of the way: Integrins in cancer progression and metastasis. Nat. Rev. Drug Discov. 2018, 17, 31–46. [Google Scholar] [CrossRef] [PubMed]
- Gao, L.; Zhang, C.; Gao, D.; Liu, H.; Yu, X.; Lai, J.; Wang, F.; Lin, J.; Liu, Z. Enhanced Anti-Tumor Efficacy through a Combination of Integrin αvβ6-Targeted Photodynamic Therapy and Immune Checkpoint Inhibition. Theranostics 2016, 6, 627–637. [Google Scholar] [CrossRef]
- Pacheco-Soares, C.; Maftou-Costa, M.; Costa, C.G.D.C.M.; Silva, A.C.D.S.; Moraes, K.C.M. Evaluation of photodynamic therapy in adhesion protein expression. Oncol. Lett. 2014, 8, 714–718. [Google Scholar] [CrossRef] [PubMed]
- Runnels, J.M.; Chen, N.; Ortel, B.; Kato, D.; Hasan, T. BPD-MA-mediated photosensitization in vitro and in vivo: Cellular adhesion and β1 integrin expression in ovarian cancer cells. Br. J. Cancer 1999, 80, 946–953. [Google Scholar] [CrossRef]
- Laklai, H.; Miroshnikova, Y.A.; Pickup, M.W.; Collisson, E.A.; Kim, G.E.; Barrett, A.S.; Hill, R.C.; Lakins, J.N.; Schlaepfer, D.D.; Mouw, J.K.; et al. Genotype tunes pancreatic ductal adenocarcinoma tissue tension to induce matricellular fibrosis and tumor progression. Nat. Med. 2016, 22, 497–505. [Google Scholar] [CrossRef] [PubMed]
- Pickup, M.W.; Mouw, J.K.; Weaver, V.M. The extracellular matrix modulates the hallmarks of cancer. EMBO Rep. 2014, 15, 1243–1253. [Google Scholar] [CrossRef] [PubMed]
- Nguyen, A.V.; Nyberg, K.D.; Scott, M.B.; Welsh, A.M.; Nguyen, A.H.; Wu, N.; Hohlbauch, S.V.; Geisse, N.A.; Gibb, E.A.; Robertson, A.G.; et al. Stiffness of pancreatic cancer cells is associated with increased invasive potential. Integr. Biol. 2016, 8, 1232–1245. [Google Scholar] [CrossRef]
- Rice, A.J.; Cortes, E.; Lachowski, D.; Cheung, B.C.H.; Karim, S.A.; Morton, J.; Hernández, A.D.R. Matrix stiffness induces epithelial–mesenchymal transition and promotes chemoresistance in pancreatic cancer cells. Oncogenesis 2017, 6, e352. [Google Scholar] [CrossRef] [PubMed]
- Cramer, G.M.; Jones, D.P.; El-Hamidi, H.; Celli, J.P. ECM Composition and Rheology Regulate Growth, Motility, and Response to Photodynamic Therapy in 3D Models of Pancreatic Ductal Adenocarcinoma. Mol. Cancer Res. 2017, 15, 15–25. [Google Scholar] [CrossRef]
- Nguyen-Ngoc, K.-V.; Cheung, K.J.; Brenot, A.; Shamir, E.; Gray, R.; Hines, W.; Yaswen, P.; Werb, Z.; Ewald, A.J. ECM microenvironment regulates collective migration and local dissemination in normal and malignant mammary epithelium. Proc. Natl. Acad. Sci. USA 2012, 109, E2595–E2604. [Google Scholar] [CrossRef]
- Shah, A.N.; Summy, J.M.; Zhang, J.; Park, S.I.; Parikh, N.U.; Gallick, G.E. Development and Characterization of Gemcitabine-Resistant Pancreatic Tumor Cells. Ann. Surg. Oncol. 2007, 14, 3629–3637. [Google Scholar] [CrossRef]
- Zhang, F.; Wu, Q.; Liu, H. NIR light-triggered nanomaterials-based prodrug activation towards cancer therapy. Wiley Interdiscip. Rev. Nanomed. Nanobiotechnol. 2020, 12, e1643. [Google Scholar] [CrossRef] [PubMed]
- Celli, J.P.; Solban, N.; Liang, A.; Pereira, S.P.; Hasan, T. Verteporfin-based photodynamic therapy overcomes gemcitabine insensitivity in a panel of pancreatic cancer cell lines. Lasers Surg. Med. 2011, 43, 565–574. [Google Scholar] [CrossRef]
- Wang, Y.; Wang, H.; Zhou, L.; Lu, J.; Jiang, B.; Liu, C.; Guo, J. Photodynamic therapy of pancreatic cancer: Where have we come from and where are we going? Photodiagnosis Photodyn. Ther. 2020, 31, 101876. [Google Scholar] [CrossRef] [PubMed]
- Hanahan, D.; Weinberg, R.A. Hallmarks of Cancer: The Next Generation. Cell 2011, 144, 646–674. [Google Scholar] [CrossRef]
- Kessel, D.; Reiners, J.J., Jr. Apoptosis and autophagy after mitochondrial or endoplasmic reticulum photodamage. Photochem. Photobiol. 2007, 83, 1024–1028. [Google Scholar] [CrossRef]
- N’Guessan, K.F.; Davis, H.W.; Chu, Z.; Vallabhapurapu, S.D.; Lewis, C.S.; Franco, R.S.; Olowokure, O.; Ahmad, S.A.; Yeh, J.J.; Bogdanov, V.Y.; et al. Enhanced Efficacy of Combination of Gemcitabine and Phosphatidylserine-Targeted Nanovesicles against Pancreatic Cancer. Mol. Ther. 2020, 28, 1876–1886. [Google Scholar] [CrossRef]
- Xie, Q.; Jia, L.; Liu, Y.-H.; Wei, C.-G. Synergetic anticancer effect of combined gemcitabine and photodynamic therapy on pancreatic cancer in vivo. World J. Gastroenterol. 2009, 15, 737–741. [Google Scholar] [CrossRef]
- Broekgaarden, M.; Rizvi, I.; Bulin, A.-L.; Petrovic, L.; Goldschmidt, R.; Massodi, I.; Celli, J.P.; Hasan, T. Neoadjuvant photodynamic therapy augments immediate and prolonged oxaliplatin efficacy in metastatic pancreatic cancer organoids. Oncotarget 2018, 9, 13009–13022. [Google Scholar] [CrossRef]
- Rizvi, I.; Celli, J.P.; Evans, C.L.; Abu-Yousif, A.O.; Muzikansky, A.; Pogue, B.; Finkelstein, D.; Hasan, T. Synergistic Enhancement of Carboplatin Efficacy with Photodynamic Therapy in a Three-Dimensional Model for Micrometastatic Ovarian Cancer. Cancer Res. 2010, 70, 9319–9328. [Google Scholar] [CrossRef]
- Anbil, S.; Rizvi, I.; Celli, J.P.; Alagic, N.; Pogue, B.W.; Hasan, T. Impact of treatment response metrics on photodynamic therapy planning and outcomes in a three-dimensional model of ovarian cancer. J. Biomed. Opt. 2013, 18, 098004. [Google Scholar] [CrossRef]
- Zuluaga, M.F.; Lange, N. Combination of photodynamic therapy with anti-cancer agents. Curr. Med. Chem. 2008, 15, 1655–1673. [Google Scholar] [CrossRef] [PubMed]
- Broekgaarden, M.; Anbil, S.; Bulin, A.-L.; Obaid, G.; Mai, Z.; Baglo, Y.; Rizvi, I.; Hasan, T. Modulation of redox metabolism negates cancer-associated fibroblasts-induced treatment resistance in a heterotypic 3D culture platform of pancreatic cancer. Biomaterials 2019, 222, 119421. [Google Scholar] [CrossRef] [PubMed]
- Celli, J.P.; Rizvi, I.; Blanden, A.R.; Massodi, I.; Glidden, M.D.; Pogue, B.; Hasan, T. An imaging-based platform for high-content, quantitative evaluation of therapeutic response in 3D tumour models. Sci. Rep. 2014, 4, 3751. [Google Scholar] [CrossRef] [PubMed]
- Anbil, S.; Pigula, M.; Huang, H.-C.; Mallidi, S.; Broekgaarden, M.; Baglo, Y.; De Silva, P.; Simeone, D.M.; Mino-Kenudson, M.; Maytin, E.V.; et al. Vitamin D Receptor Activation and Photodynamic Priming Enables Durable Low-dose Chemotherapy. Mol. Cancer Ther. 2020, 19, 1308–1319. [Google Scholar] [CrossRef]
- Zhang, F.; Han, X.; Hu, Y.; Wang, S.; Liu, S.; Pan, X.; Wang, H.; Ma, J.; Wang, W.; Li, S.; et al. Interventional Photothermal Therapy Enhanced Brachytherapy: A New Strategy to Fight Deep Pancreatic Cancer. Adv. Sci. 2019, 6, 1801507. [Google Scholar] [CrossRef]
- Shunhao, W.; Chi, C.; Wang, S.; Wang, L.; Liang, P.; Liu, F.; Shang, W.; Wang, W.; Zhang, F.; Lingxiong, W.; et al. A Comparative Study of Clinical Intervention and Interventional Photothermal Therapy for Pancreatic Cancer. Adv. Mater. 2017, 29, 1700448. [Google Scholar] [CrossRef]
- Li, H.; Wang, P.; Deng, Y.; Zeng, M.; Tang, Y.; Zhu, W.-H.; Cheng, Y. Combination of active targeting, enzyme-triggered release and fluorescent dye into gold nanoclusters for endomicroscopy-guided photothermal/photodynamic therapy to pancreatic ductal adenocarcinoma. Biomaterials 2017, 139, 30–38. [Google Scholar] [CrossRef]
- Li, W.; Zhang, H.; Guo, X.; Wang, Z.; Kong, F.; Luo, L.; Li, Q.; Zhu, C.; Yang, J.; Lou, Y.; et al. Gold Nanospheres-Stabilized Indocyanine Green as a Synchronous Photodynamic–Photothermal Therapy Platform That Inhibits Tumor Growth and Metastasis. ACS Appl. Mater. Interfaces 2017, 9, 3354–3367. [Google Scholar] [CrossRef]
- Vankayala, R.; Huang, Y.-K.; Kalluru, P.; Chiang, C.-S.; Hwang, K.C. First Demonstration of Gold Nanorods-Mediated Photodynamic Therapeutic Destruction of Tumors via Near Infra-Red Light Activation. Small 2014, 10, 1612–1622. [Google Scholar] [CrossRef]
- Li, Y.J.; Huang, P.; Jiang, C.L.; Jia, D.X.; Du, X.X.; Zhou, J.H.; Han, Y.; Sui, H.; Wei, X.L.; Liu, L.; et al. Sonodynamically Induced Anti-tumor Effect of 5-Aminolevulinic Acid on Pancreatic Cancer Cells. Ultrasound Med. Biol. 2014, 40, 2671–2679. [Google Scholar] [CrossRef]
- Sun, H.; Zhang, Y.; Chen, S.; Wang, R.; Chen, Q.; Li, J.; Luo, Y.; Wang, X.; Chen, H. Photothermal Fenton Nanocatalysts for Synergetic Cancer Therapy in the Second Near-Infrared Window. ACS Appl. Mater. Interfaces 2020, 12, 30145–30154. [Google Scholar] [CrossRef] [PubMed]
- Ni, K.; Aung, T.; Li, S.; Fatuzzo, N.; Liang, X.; Lin, W. Nanoscale Metal-Organic Framework Mediates Radical Therapy to Enhance Cancer Immunotherapy. Chem 2019, 5, 1892–1913. [Google Scholar] [CrossRef] [PubMed]
- Zhou, Y.; Wang, M.; Dai, Z. The molecular design of and challenges relating to sensitizers for cancer sonodynamic therapy. Mater. Chem. Front. 2020, 4, 2223–2234. [Google Scholar] [CrossRef]
- Chen, J.; Luo, H.; Liu, Y.; Zhang, W.; Li, H.; Luo, T.; Zhang, K.; Zhao, Y.; Liu, J. Oxygen-Self-Produced Nanoplatform for Relieving Hypoxia and Breaking Resistance to Sonodynamic Treatment of Pancreatic Cancer. ACS Nano 2017, 11, 12849–12862. [Google Scholar] [CrossRef] [PubMed]
- Blum, N.T.; Zhang, Y.; Qu, J.; Lin, J.; Huang, P. Recent Advances in Self-Exciting Photodynamic Therapy. Front. Bioeng. Biotechnol. 2020, 8, 594491. [Google Scholar] [CrossRef]
- Clement, S.; Anwer, A.; Deng, W.; Wilson, B.C.; Allen, C.; Goldys, E.M. Nuclear targeted x-ray activated photodynamic therapy: A solution to treat pancreatic cancer (Conference Presentation). Opt. Methods Tumor Treat. Detect. Mech. Tech. Photodyn. Therapy XXVIII 2019, 10860, 1086009. [Google Scholar] [CrossRef]
- Cline, B.; Delahunty, I.; Xie, J. Nanoparticles to mediate X-ray-induced photodynamic therapy and Cherenkov radiation photodynamic therapy. Wiley Interdiscip. Rev. Nanomed. Nanobiotechnol. 2019, 11, e1541. [Google Scholar] [CrossRef]
- Chen, W.; Zhang, J. Using Nanoparticles to Enable Simultaneous Radiation and Photodynamic Therapies for Cancer Treatment. J. Nanosci. Nanotechnol. 2006, 6, 1159–1166. [Google Scholar] [CrossRef]
- Bulin, A.-L.; Broekgaarden, M.; Simeone, D.; Hasan, T. Low dose photodynamic therapy harmonizes with radiation therapy to induce beneficial effects on pancreatic heterocellular spheroids. Oncotarget 2019, 10, 2625–2643. [Google Scholar] [CrossRef]
- Anand, S.; Chan, T.; Hasan, T.; Maytin, E. Current Prospects for Treatment of Solid Tumors via Photodynamic, Photothermal, or Ionizing Radiation Therapies Combined with Immune Checkpoint Inhibition (A Review). Pharmaceuticals 2021, 14, 447. [Google Scholar] [CrossRef]
- Sun, F.; Zhu, Q.; Li, T.; Saeed, M.; Xu, Z.; Zhong, F.; Song, R.; Huai, M.; Zheng, M.; Xie, C.; et al. Regulating Glucose Metabolism with Prodrug Nanoparticles for Promoting Photoimmunotherapy of Pancreatic Cancer. Adv. Sci. 2021, 8, 2002746. [Google Scholar] [CrossRef]
- Kleinovink, J.W.; Van Driel, P.B.; Snoeks, T.J.; Prokopi, A.; Fransen, M.F.; Cruz, L.J.; Mezzanotte, L.; Chan, A.; Löwik, C.W.; Ossendorp, F. Combination of Photodynamic Therapy and Specific Immunotherapy Efficiently Eradicates Established Tumors. Clin. Cancer Res. 2016, 22, 1459–1468. [Google Scholar] [CrossRef]
- Lu, J.; Roy, B.; Anderson, M.; Leggett, C.L.; Levy, M.J.; Pogue, B.; Hasan, T.; Wang, K.K. Verteporfin- and sodium porfimer-mediated photodynamic therapy enhances pancreatic cancer cell death without activating stromal cells in the microenvironment. J. Biomed. Opt. 2019, 24, 118001. [Google Scholar] [CrossRef] [PubMed]
- Celli, J.P. Stromal Interactions as Regulators of Tumor Growth and Therapeutic Response: A Potential Target for Photodynamic Therapy? Isr. J. Chem. 2012, 52, 757–766. [Google Scholar] [CrossRef] [PubMed]
- Jafari, R.; Cramer, G.M.; Celli, J.P. Modulation of Extracellular Matrix Rigidity Via Riboflavin-mediated Photocrosslinking Regulates Invasive Motility and Treatment Response in a 3D Pancreatic Tumor Model. Photochem. Photobiol. 2019, 96, 365–372. [Google Scholar] [CrossRef] [PubMed]
- Gilles, M.-E.; Hao, L.; Huang, L.; Rupaimoole, R.; López-Casas, P.P.; Pulver, E.; Jeong, J.C.; Muthuswamy, S.K.; Hidalgo, M.; Bhatia, S.N.; et al. Personalized RNA Medicine for Pancreatic Cancer. Clin. Cancer Res. 2018, 24, 1734–1747. [Google Scholar] [CrossRef] [PubMed]
- Sicard, F.; Gayral, M.; Lulka, H.; Buscail, L.; Cordelier, P. Targeting miR-21 for the Therapy of Pancreatic Cancer. Mol. Ther. 2013, 21, 986–994. [Google Scholar] [CrossRef] [PubMed]
- Zhao, G.; Wang, B.; Liu, Y.; Zhang, J.-G.; Deng, S.-C.; Qin, Q.; Tian, K.; Li, X.; Zhu, S.; Niu, Y.; et al. miRNA-141, Downregulated in Pancreatic Cancer, Inhibits Cell Proliferation and Invasion by Directly Targeting MAP4K4. Mol. Cancer Ther. 2013, 12, 2569–2580. [Google Scholar] [CrossRef]
- Tu, M.-J.; Pan, Y.-Z.; Qiu, J.-X.; Kim, E.J.; Yu, A. MicroRNA-1291 targets the FOXA2-AGR2 pathway to suppress pancreatic cancer cell proliferation and tumorigenesis. Oncotarget 2016, 7, 45547–45561. [Google Scholar] [CrossRef] [PubMed]
- Muller, S.; Raulefs, S.; Bruns, P.; Afonso-Grunz, F.; Plötner, A.; Thermann, R.; Jäger, C.; Schlitter, A.M.; Kong, B.; Regel, I.; et al. Next-generation sequencing reveals novel differentially regulated mRNAs, lncRNAs, miRNAs, sdRNAs and a piRNA in pancreatic cancer. Mol. Cancer 2015, 14, 1–18. [Google Scholar] [CrossRef]
- Rachagani, S.; Macha, M.; Menning, M.S.; Dey, P.; Pai, P.; Smith, L.M.; Mo, Y.-Y.; Batra, S.K. Changes in microRNA (miRNA) expression during pancreatic cancer development and progression in a genetically engineered KrasG12D;Pdx1-Cre mouse (KC) model. Oncotarget 2015, 6, 40295–40309. [Google Scholar] [CrossRef]
- Medina, P.P.; Nolde, M.J.; Slack, F. OncomiR addiction in an in vivo model of microRNA-21-induced pre-B-cell lymphoma. Nat. Cell Biol. 2010, 467, 86–90. [Google Scholar] [CrossRef] [PubMed]
- Li, Y.; Sarkar, F.H. MicroRNA Targeted Therapeutic Approach for Pancreatic Cancer. Int. J. Biol. Sci. 2016, 12, 326–337. [Google Scholar] [CrossRef] [PubMed]
- Dong, J.; Zhao, Y.-P.; Zhou, L.; Zhang, T.-P.; Chen, G. Bcl-2 Upregulation Induced by miR-21 Via a Direct Interaction Is Associated with Apoptosis and Chemoresistance in MIA PaCa-2 Pancreatic Cancer Cells. Arch. Med. Res. 2011, 42, 8–14. [Google Scholar] [CrossRef]
- Kessel, D.; Castelli, M. Evidence that bcl-2 is the Target of Three Photosensitizers that Induce a Rapid Apoptotic Response¶. Photochem. Photobiol. 2007, 74, 318–322. [Google Scholar] [CrossRef]
- Wang, H.-L.; Zhou, R.; Liu, J.; Chang, Y.; Liu, S.; Wang, X.-B.; Huang, M.-F.; Zhao, Q. MicroRNA-196b inhibits late apoptosis of pancreatic cancer cells by targeting CADM1. Sci. Rep. 2017, 7, 11467. [Google Scholar] [CrossRef]
Publisher’s Note: MDPI stays neutral with regard to jurisdictional claims in published maps and institutional affiliations.
|
© 2021 by the authors. Licensee MDPI, Basel, Switzerland. This article is an open access article distributed under the terms and conditions of the Creative Commons Attribution (CC BY) license (https://creativecommons.org/licenses/by/4.0/).
Gerelateerde artikelen
- Interstitiële fotodynamische therapie (iPDT) met 5-aminolevulinezuur aanvullend aan standaard behandeling geeft bij operabele Glioblastoma langere progressievrije ziekte en overall overleving in vergelijking met operatie plus alleen chemo en bestraling co
- Photo Immuno Therapy (PIT) = PDT met infrarood licht blijkt veelbelovende vorm van immuuntherapie voor solide tumoren al of niet in combinatie met andere behandelingen copy 1
- Curcumine gecombineerd met PDT - Foto Dynamische Therapie geeft uitstekende resultaten bij verschillende vormen van kanker blijkt uit recent gepubliceerde reviewstudie copy 1
- PDT - Foto Dynamische Therapie bij alvleesklierkanker kan zinvol zijn, zeker in combinatie met andere behandelingen, waaronder zelfs immuuntherapie, blijkt uit reviewstudie
- Laserwatch met capsules bremachlorofyl voor thuis zelf PDT geven op de bloedvaten om uitzaaiingen of een recidief te voorkomen zijn via ons te bestellen.
- PDT - Foto Dynamische Therapie op in bloed circulerende tumorcellen toe passen op het bloed in een extracorporale buis heeft groot effect op doden van tumorcellen.
- PDT - Fotodynamische therapie vermindert kans op uitzaaiingen van borstkanker doordat er veel minder in bloed circulerende tumorcellen zijn.
- PDT - Foto Dynamische Therapie gebruikt bij kankerpatienten stimuleert immuunsysteem en is soms succesvol als immuuntherapie. Hier een overzichtstudie
- Onderzoekers van de Technische Universiteit van Eindhoven verbeteren PDT - fotodynamische therapie met behulp van nanotechnologie. Een doorbraak in kankerchirurgie
- PDT - Photo Dynamische Therapie op in bloed circulerende tumorcellen lijkt met nieuwe techniek in korte tijd alle tumorcellen te doden en kan daarmee uitzaaiingen en recidieven voorkomen
- Algemeen: PDT - Photodynamische Therapie (laser- of lichttherapie) in Duitsland met enkele vragen en antwoorden over wat PDT precies inhoudt
- Algemeen: PDT - Photo Dynamische Therapie activeert ingebracht virus en lijkt een nieuwe mogelijke PDT behandelings optie variant.
- Algemeen: Nederlands Kanker Instituut opende in 2006 nieuw behandelcentrum lichttherapie voor bijna alle vormen van kanker.
- Alvleesklierkanker: PDT - Fotodynamische therapie succesvol bij alvleesklierpatiënten. overzichtstudie toegevoegd..
- Blaaskanker: PDT - fotodynamische therapie toont significant beter effect bij blaaskanker en recidief van blaaskanker. Overzichtstudie toegevoegd
- Borstkanker: PDT - Photodynamische therapie ook succesvol bij borstkankerpatiënten bij recidief na operatie aldus verschillende studies.
- Dendritische celtherapie: Een combinatie van lokale PDT - Photo Dynamische Therapie gevolgd door dendritische celtherapie zorgt bij muizen voor opmerkelijk veel remissies waar de afzonderlijke behandelingen niets deden.
- Photosynthesizer bij PDT - talaporfin sodium - gemaakt van chlorophyl uit planten door FDA goedgekeurd voor gebruik bij PDT.
- Hersentumoren: PDT - Photodynamische Therapie met ALA en Fotofrin en soms ook herhaald verdubbelt ziektevrije tijd, verbetert significant levensduur en kwaliteit van leven bij hersentumoren - Glioblastoma Multiforme.
- Hoofd- en halstumoren: PDT - Photodynamische Therapie blijkt succesvolle aanpak van hoofd-halstumoren stadium 1 2 en 3, blijkt uit Nederlandse studie
- Huidkanker: PDT - Photodynamische therapie ook effectief bij vormen van huidkanker waarbij een bacteriële infectie de oorzaak is. Artikel update 1 december 2011
- Levertumoren: PDT - Photodynamische therapie toont mooi effect bij 5 patiënten met levermetastases vanuit darmkanker.
- Longkanker: PDT - Photodynamische therapie met foscan geeft succes bij mesothelioma, (asbestkanker) aldus fase I studie.
- Nanotechnologie in combinatie met PDT - Photo Dynamische Therapie is een veelbelovende behandelingstechniek voor kanker
- Prostaatkanker: PDT - Photo Dynamische Therapie op de bloedvaten bij prostaatkankerpatienten met een wait-and-see beleid geeft op 2 jaar verdubbeling 28% vs 58% van progressievrije ziekte in vergelijking met wait-and-see beleid
Plaats een reactie ...
Reageer op "PDT - Foto Dynamische Therapie bij alvleesklierkanker kan zinvol zijn, zeker in combinatie met andere behandelingen, waaronder zelfs immuuntherapie, blijkt uit reviewstudie"