rekeningnummer RABO 37.29.31.138 t.n.v. Stichting Gezondheid Actueel in Terneuzen.
Onze IBANcode is NL79 RABO 0372 9311 38 BIC/SWIFTCODE RABONL2U
12 februari 2024: Bron: Nature
Veelbelovende resultaten voor het specifiek samengestelde kankervaccin ELI-002 2P worden gezien in de fase I veiligheidsstudie bij 25 deelnemende patiënten, waarvan 20 met alvleesklierkanker en 5 met endeldarmkanker, allemaal met een KRAS positieve mutatie.
In deze fase I studie werden 20 patiënten met KRAS-gemuteerde alvleesklierkanker en 5 patiënten met endeldarmkanker behandelt met een locoregionale behandeling met het kankervaccin ELI-002 2P. Er werden geen dosisbeperkende bijwerkingen waargenomen.
De tumorbiomarkerrespons, het secundaire eindpunt, werd waargenomen bij 84% van de patiënten, terwijl de biomarkerklaring naar normale waarden werd waargenomen bij 24%, 3 patiënten met alvleesklierkanker en 3 patiënten met endeldarmkanker. De mediane recidiefvrije overleving was 16,33 maanden.
Uit het studieverslag hier het schema van toediening in 5 verschillende groepen en hoe de patiënten werden gekozen:
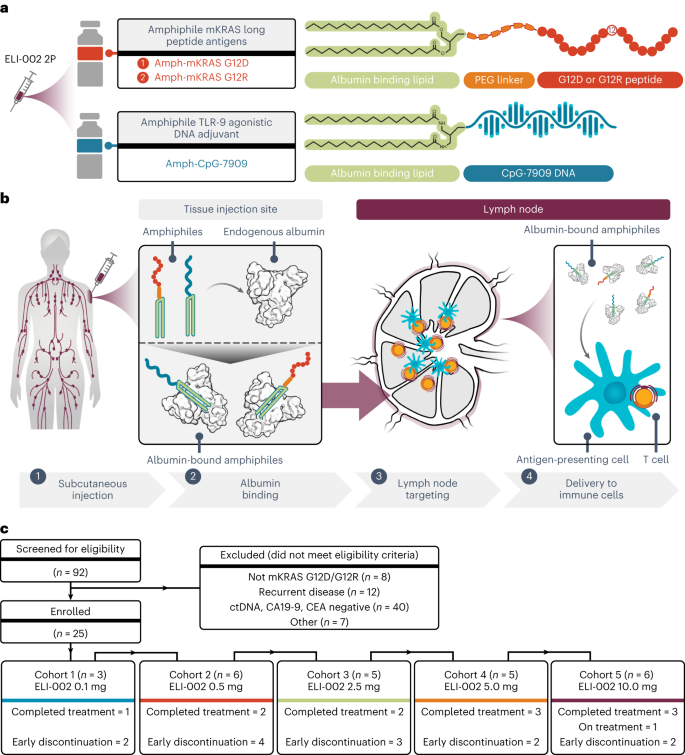
a, Schematic for ELI-002 2P vaccine components, including Amph-mKRAS G12D and G12R long peptide antigens and Amph-CpG-7909 TLR9 agonist. PEG, polyethylene glycol. b, Stepwise schematic for Amph-directed lymph-node-targeted biodistribution mechanism using albumin ‘hitchhiking’: (1) subcutaneous Amph injection, followed by (2) lipid-mediated non-covalent molecular association of Amph vaccines with tissue-resident endogenous albumin, resulting in (3) preferential absorption into lymphatics and accumulation through afferent lymph flow into draining lymph nodes and, finally, (4) uptake of Amph vaccines by lymph-node-resident APCs to induce antigen presentation and coordinated co-stimulation of cognate T cells. c, CONSORT diagram. Patients were enrolled into five successive cohorts with progressively increasing doses of Amph-CpG-7909 with a fixed dose of Amph-Peptides 2P. Graphical elements from a and b were adapted from previous publications under a Creative Commons Attribution 4.0 International License (http://creativecommons.org/licenses/by/4.0/) (refs. 57,58).
Alvleesklierkanker en vormen van darmkanker zijn vaak KRAS-gemuteerd en zijn meestal ongeneeslijk wanneer er een recidief optreedt met dezelfde tumor-DNA mutatie of tumoreiwit expressie na een (chemo) behandeling met als doel genezing.
Uit dierstudies is gebleken dat het kankervaccin ELI-002 2P de behandeling verbetert bij uitzaaiingen in de lymfeklieren en de immuunrespons met behulp van amfifiele (Amph) modificatie van G12D- en G12R-mutante KRAS (mKRAS) peptiden (Amph-Peptides-2P) indien gegeven samen met Amph-CpG-7909 (CpG-oligonucleotide-adjuvans).
De onderzoekers van de studie zagen deze onderstaande resultaten, zie grafieken onder Fig. 3a en uitgebreide data Fig. 3:
- Directe ex vivo mKRAS-specifieke T-celreacties werden waargenomen bij 21 van de 25 patiënten (84%; 59% zowel CD4+ als CD8+);
- tumorbiomarkerreacties werden waargenomen bij 21 van de 25 patiënten (84%);
- klaring van biomarkers werd waargenomen bij zes van de 25 patiënten (24%; drie alvleesklierkankerpatiënten en drie darmkankerpatiënten);
- en de mediane recidiefvrije tijd (RFS) was 16,33 maanden.
- De werkzaamheid correleerde met T-celresponsen boven of onder de mediaan-voudige toename ten opzichte van de uitgangswaarde (12,75-voudig): de mediane reductie van de tumorbiomarker was -76,0% versus -10,2% (P < 0,0014), en de mediane recidiefvrije tijd (RFS) werd niet bereikt versus 4,01 maanden ( risicoratio = 0,14; P = 0,0167).
De conclusie van de onderzoekers: ELI-002 2P was veilig en stimuleerde aanzienlijke T-celreacties bij patiënten met voor immuuntherapie ongevoelige KRAS-gemuteerde tumoren.
(Fase II gerandomiseerde studie is reeds gestart met de volgende KRAS mutaties: G12D, G12R, G12V, G12A, G12C, G12S, G13D). Zie dit studieprotocol
Het originele studierapport van de fase I studie is in Nature gepubliceerd en vrij in te zien of te downloaden:
- Article
- Open access
- Published:
Lymph-node-targeted, mKRAS-specific amphiphile vaccine in pancreatic and colorectal cancer: the phase 1 AMPLIFY-201 trial
Nature Medicine (2024)
Abstract
Pancreatic and colorectal cancers are often KRAS mutated and are incurable when tumor DNA or protein persists or recurs after curative intent therapy. Cancer vaccine ELI-002 2P enhances lymph node delivery and immune response using amphiphile (Amph) modification of G12D and G12R mutant KRAS (mKRAS) peptides (Amph-Peptides-2P) together with CpG oligonucleotide adjuvant (Amph-CpG-7909). We treated 25 patients (20 pancreatic and five colorectal) who were positive for minimal residual mKRAS disease (ctDNA and/or serum tumor antigen) after locoregional treatment in a phase 1 study of fixed-dose Amph-Peptides-2P and ascending-dose Amph-CpG-7909; study enrollment is complete with patient follow-up ongoing. Primary endpoints included safety and recommended phase 2 dose (RP2D). The secondary endpoint was tumor biomarker response (longitudinal ctDNA or tumor antigen), with exploratory endpoints including immunogenicity and relapse-free survival (RFS). No dose-limiting toxicities were observed, and the RP2D was 10.0 mg of Amph-CpG-7909. Direct ex vivo mKRAS-specific T cell responses were observed in 21 of 25 patients (84%; 59% both CD4+ and CD8+); tumor biomarker responses were observed in 21 of 25 patients (84%); biomarker clearance was observed in six of 25 patients (24%; three pancreatic and three colorectal); and the median RFS was 16.33 months. Efficacy correlated with T cell responses above or below the median fold increase over baseline (12.75-fold): median tumor biomarker reduction was −76.0% versus −10.2% (P < 0.0014), and the median RFS was not reached versus 4.01 months (hazard ratio = 0.14; P = 0.0167). ELI-002 2P was safe and induced considerable T cell responses in patients with immunotherapy-recalcitrant KRAS-mutated tumors. ClinicalTrials.gov identifier: NCT04853017.
Data availability
Requests must be made to datarequest@elicio.com, with responses provided within 30 d of request. To ensure that data sharing is consistent with the underlying study consent, de-identified patient data that can be shared will be done under data transfer agreements. Investigators and institutions who agree to the terms of the data transfer agreement, which will include, but will not be limited to, terms to address the use of these data for the purposes of a specific project and for research purposes only, to prohibit attempts to re-identify the data and to protect the confidentiality of the data, will be granted access to the data. Elicio Therapeutics will then facilitate the transfer of the requested de-identified data to the requestor using secure electronic data transmission. The data will then be available for up to 12 months.
References
-
Siegel, R. L., Miller, K. D., Fuchs, H. E. & Jemal, A. Cancer statistics, 2021. CA Cancer J. Clin. 71, 7–33 (2021).
-
Siegel, R. L., Miller, K. D. & Jemal, A. Cancer statistics, 2020. CA Cancer J. Clin. 70, 7–30 (2020).
-
Park, W., Chawla, A. & O’Reilly, E. M. Pancreatic cancer. JAMA 326, 851 (2021).
-
Modest, D. P., Pant, S. & Sartore-Bianchi, A. Treatment sequencing in metastatic colorectal cancer. Eur. J. Cancer 109, 70–83 (2019).
-
Royal, R. E. et al. Phase 2 trial of single agent ipilimumab (anti-CTLA-4) for locally advanced or metastatic pancreatic adenocarcinoma. J. Immunother. 33, 828–833 (2010).
-
Le, D. T. et al. PD-1 blockade in tumors with mismatch-repair deficiency. N. Engl. J. Med. 372, 2509–2520 (2015).
-
Brahmer, J. R. et al. Safety and activity of anti–PD-L1 antibody in patients with advanced cancer. N. Engl. J. Med. 366, 2455–2465 (2012).
-
O’Reilly, E. M. et al. Durvalumab with or without tremelimumab for patients with metastatic pancreatic ductal adenocarcinoma. JAMA Oncol. 5, 1431 (2019).
-
Biankin, A. V. et al. Pancreatic cancer genomes reveal aberrations in axon guidance pathway genes. Nature 491, 399–405 (2012).
-
Prior, I. A., Lewis, P. D. & Mattos, C. A comprehensive survey of Ras mutations in cancer. Cancer Res. 72, 2457–2467 (2012).
-
Leidner, R. et al. Neoantigen T-cell receptor gene therapy in pancreatic cancer. N. Engl. J. Med. 386, 2112–2119 (2022).
-
Tran, E. et al. T-cell transfer therapy targeting mutant KRAS in cancer. N. Engl. J. Med. 375, 2255–2262 (2016).
-
Ino, Y. et al. Immune cell infiltration as an indicator of the immune microenvironment of pancreatic cancer. Br. J. Cancer 108, 914–923 (2013).
-
Trevaskis, N. L., Kaminskas, L. M. & Porter, C. J. H. From sewer to saviour-targeting the lymphatic system to promote drug exposure and activity. Nat. Rev. Drug Discov. 14, 781–803 (2015).
-
Liu, H. et al. Structure-based programming of lymph-node targeting in molecular vaccines. Nature 507, 519–522 (2014).
-
Supersaxo, A., Hein, W. R. & Steffen, H. Effect of molecular weight on the lymphatic absorption of water-soluble compounds following subcutaneous administration. Pharm. Res. 07, 167–169 (1990).
-
McLennan, D. N., Porter, C. J. H. & Charman, S. A. Subcutaneous drug delivery and the role of the lymphatics. Drug Discov. Today Technol. 2, 89–96 (2005).
-
Seenappa, L. M. et al. Amphiphile-CpG vaccination induces potent lymph node activation and COVID-19 immunity in mice and non-human primates. NPJ Vaccines 7, 128 (2022).
-
Ma, L. et al. Vaccine-boosted CAR T crosstalk with host immunity to reject tumors with antigen heterogeneity. Cell 186, 3148–3165 (2023).
-
Martin, J. T. et al. Combined PET and whole-tissue imaging of lymphatic-targeting vaccines in non-human primates. Biomaterials 275, 120868 (2021).
-
Moynihan, K. D. et al. Enhancement of peptide vaccine immunogenicity by increasing lymphatic drainage and boosting serum stability. Cancer Immunol. Res. 6, 1025–1038 (2018).
-
Ryschich, E. et al. Control of T-cell-mediated immune response by HLA class I in human pancreatic carcinoma. Clin. Cancer Res. 11, 498–504 (2005).
-
Baleeiro, R. B. et al. Optimized anchor-modified peptides targeting mutated RAS are promising candidates for immunotherapy. Front. Immunol. 13, 902709 (2022).
-
Sim, M. J. W. et al. High-affinity oligoclonal TCRs define effective adoptive T cell therapy targeting mutant KRAS-G12D. Proc. Natl Acad. Sci. USA 117, 12826–12835 (2020).
-
Qin, H. et al. CD4+ T-cell immunity to mutated Ras protein in pancreatic and colon cancer patients. Cancer Res. 55, 2984–2987 (1995).
-
Cafri, G. et al. Memory T cells targeting oncogenic mutations detected in peripheral blood of epithelial cancer patients. Nat. Commun. 10, 449 (2019).
-
Veatch, J. R. et al. Endogenous CD4+ T cells recognize neoantigens in lung cancer patients, including recurrent oncogenic KRAS and ERBB2 (Her2) driver mutations. Cancer Immunol. Res. 7, 910–922 (2019).
-
Tran, E. et al. Immunogenicity of somatic mutations in human gastrointestinal cancers. Science 350, 1387–1390 (2015).
-
Anderson, J. R., Cain, K. C. & Gelber, R. D. Analysis of survival by tumor response. J. Clin. Oncol. 1, 710–719 (1983).
-
Allen, P. J. et al. Multi-institutional validation study of the American Joint Commission on Cancer (8th edition) changes for T and N staging in patients with pancreatic adenocarcinoma. Ann Surg. 265, 185–191 (2017).
-
Lee, B. et al. Circulating tumor DNA as a potential marker of adjuvant chemotherapy benefit following surgery for localized pancreatic cancer. Ann. Oncol. 30, 1472–1478 (2019).
-
Groot, V. P. et al. Circulating tumor DNA as a clinical test in resected pancreatic cancer. Clin. Cancer Res. 25, 4973–4984 (2019).
-
Rieser, C. J. et al. CA19-9 on postoperative surveillance in pancreatic ductal adenocarcinoma: predicting recurrence and changing prognosis over time. Ann. Surg. Oncol. 25, 3483–3491 (2018).
-
Botta, G. P. et al. Association of personalized and tumor-informed ctDNA with patient survival outcomes in pancreatic adenocarcinoma. J. Clin. Oncol. 40, 517 (2022).
-
Reinert, T. et al. Analysis of circulating tumour DNA to monitor disease burden following colorectal cancer surgery. Gut 65, 625–634 (2016).
-
Tie, J. et al. Circulating tumor DNA analyses as markers of recurrence risk and benefit of adjuvant therapy for stage III colon cancer. JAMA Oncol. 5, 1710 (2019).
-
Kotani, D. et al. Molecular residual disease and efficacy of adjuvant chemotherapy in patients with colorectal cancer. Nat. Med. 29, 127–134 (2023).
-
Rojas, L. A. et al. Personalized RNA neoantigen vaccines stimulate T cells in pancreatic cancer. Nature 618, 144–150 (2023).
-
Zent, C. S. et al. Phase I clinical trial of CpG oligonucleotide 7909 (PF-03512676) in patients with previously treated chronic lymphocytic leukemia. Leuk. Lymphoma 53, 211–217 (2012).
-
van Meer, L. et al. Injection site reactions after subcutaneous oligonucleotide therapy. Br. J. Clin. Pharmacol. 82, 340–351 (2016).
-
Palmer, D. H. et al. TG01/GM-CSF and adjuvant gemcitabine in patients with resected RAS-mutant adenocarcinoma of the pancreas (CT TG01-01): a single-arm, phase 1/2 trial. Br. J. Cancer 122, 971–977 (2020).
-
Ott, P. A. et al. An immunogenic personal neoantigen vaccine for patients with melanoma. Nature 547, 217–221 (2017).
-
Sahin, U. et al. Personalized RNA mutanome vaccines mobilize poly-specific therapeutic immunity against cancer. Nature 547, 222–226 (2017).
-
Bear, A. S. et al. Biochemical and functional characterization of mutant KRAS epitopes validates this oncoprotein for immunological targeting. Nat. Commun. 12, 4365 (2021).
-
Poole, A. et al. Therapeutic high affinity T cell receptor targeting a KRASG12D cancer neoantigen. Nat. Commun. 13, 5333 (2022).
-
Gjertsen, M. K. et al. Intradermal ras peptide vaccination with granulocyte-macrophage colony-stimulating factor as adjuvant: clinical and immunological responses in patients with pancreatic adenocarcinoma. Int. J. Cancer 92, 441–450 (2001).
-
Carbone, D. P. et al. Immunization with mutant p53- and K-ras–derived peptides in cancer patients: immune response and clinical outcome. J. Clin. Oncol. 23, 5099–5107 (2005).
-
Palmer, C. D. et al. Individualized, heterologous chimpanzee adenovirus and self-amplifying mRNA neoantigen vaccine for advanced metastatic solid tumors: phase 1 trial interim results. Nat. Med. 28, 1619–1629 (2022).
-
Awad, M. M. et al. Acquired resistance to KRASG12C inhibition in cancer. N. Engl. J. Med. 384, 2382–2393 (2021).
-
Awad, M. M. et al. Personalized neoantigen vaccine NEO-PV-01 with chemotherapy and anti-PD-1 as first-line treatment for non-squamous non-small cell lung cancer. Cancer Cell. 40, 1010–1026 (2022).
-
Khattak, A. et al. A personalized cancer vaccine, mRNA-4157, combined with pembrolizumab versus pembrolizumab in patients with resected high-risk melanoma: efficacy and safety results from the randomized, open-label phase 2 mRNA-4157-P201/KEYNOTE-942 trial. In: Proc. of the 114th Annual Meeting of the American Association for Cancer Research. https://www.abstractsonline.com/pp8/#!/10828/presentation/10243 (American Association for Cancer Research, 2023).
-
Moynihan, K. D. et al. Eradication of large established tumors in mice by combination immunotherapy that engages innate and adaptive immune responses. Nat. Med. 22, 1402–1410 (2016).
-
Ma, L. et al. Enhanced CAR–T cell activity against solid tumors by vaccine boosting through the chimeric receptor. Science 365, 162–168 (2019).
-
Drakes, D. J. et al. Lymph node-targeted vaccine boosting of TCR-T cell therapy enhances anti-tumor function and eradicates solid tumors. Preprint at bioRxiv https://doi.org/10.1101/2022.05.05.490779 (2022).
-
Kemp, S. B. et al. Efficacy of a small-molecule inhibitor of KrasG12D in immunocompetent models of pancreatic cancer. Cancer Discov. 13, 298–311 (2023).
-
Ademmer, K. et al. Effector T lymphocyte subsets in human pancreatic cancer: detection of CD8+ CD18+ cells and CD8+ CD103+ cells by multi-epitope imaging. Clin. Exp. Immunol. 112, 21–26 (2001).
-
Dasari, V. et al. Lymph node targeted multi-epitope subunit vaccine promotes effective immunity to EBV in HLA-expressing mice. Nat. Commun. 14, 4371 (2023).
-
Steinbuck, M. P. et al. A lymph node–targeted Amphiphile vaccine induces potent cellular and humoral immunity to SARS-CoV-2. Sci Adv. 7, eabe5819 (2021).
Acknowledgements
The study was sponsored and funded by Elicio Therapeutics. We thank the patients and their caregivers and families for their participation in this study; the physicians, nurses and site staff who cared for the patients and supported the study; and D. M. Lidgate for expert medical writing assistance. Employees of Elicio Therapeutics received salaries for their contributions to the study. Aside from grants to their institutions, no other authors received specific funding for this work.
Author information
Authors and Affiliations
Contributions
C.M.H., E.W., P.C.D. and L.K.M. conceived the clinical trial concept. S.P., E.O.R., Z.W., C.W., M.F., P.K., C.D., A.L. and V.C. enrolled patients to the clinical trial, evaluated toxicity and participated in critical discussions and in manuscript writing and editing. O.B. performed the pathology assessments. H.V., A.T., L.M.S., J.R.P. and L.K.M. performed the immunological assays. L.K.M., T.K. and P.C.D. conducted formal analysis, data visualization, writing—original draft and writing—review and editing. E.W. obtained resources, managed data, performed project administration and performed writing—review and editing. P.C.D. and C.M.H contributed to writing—original draft, writing—review and editing, funding acquisition, formal analysis and data curation.
Corresponding authors
Ethics declarations
Competing interests
S.P. reports clinical research funding to institution from Arqule, Bristol Myers Squibb, Eli Lilly, Elicio Therapeutics, Holy Stone Healthcare, Ipsen, Mirati Therapeutics, Novartis, Rgenix, Sanofi-Aventis, Xencor, Astellas, Framewave, 4D Pharma, Boehringer Ingelheim, NGM Biopharmaceuticals, Janssen, Arcus Biosciences, BioNTech, Zymeworks and Pfizer. S.P. also reports consultant/advisory fees from Zymeworks, Ipsen, Novartis, Janssen and Boehringer Ingelheim. Z.A.W. has received consultant/advisory fees from Lilly Oncology, AstraZeneca, Merck, Daiichi Sankyo, Macrogenics, Amgen, Bristol Myers Squibb, Astellas, Ipsen, Arcus, Novartis, Roche, Seagen and Pfizer and has received research funding (institutional) from Elicio Therapeutics, Arcus, Plexxikon, Novartis and Merck. C.D.W. reports research funding from Elicio Therapeutics, Novartis, Actuate Therapeutics, Merck, AstraZeneca and Mirati and advisory board relationships with Ipsen, Actuate Therapeutics and Genentech. M.F. has received research funding (institutional) from AstraZeneca, Bristol Myers Squibb, Eli Lilly, Merck, Novartis, Pfizer, Roche, Genmab, Elicio Therapeutics, Mirati, Amgen, Replimmune, Checkmate Pharmaceuticals, Gilead, GlaxoSmithKline, Immunocore, Seagen, Tesaro and Abbvie and has been on advisory boards for Abbvie, Beigene, Jazz Pharmaceuticals, Mirati and AstraZeneca. P.M.K. reports a consultancy/advisory board relationship with Elicio (scientific advisory board member/stock ownership); consultancy/advisory board relationships with Guardant Health, Illumina, Natera, Foundation Medicine, Daichi Sankyo, Tempus, Bayer, MSD Oncology/Merck, Delcath Systems, QED Therapeutics, Taiho Oncology (self/institution), Exact Sciences, Eisai, BostonGene, Neogenomics, Saga Diagnostics, Servier, Seagen, Eli Lilly and Ipsen (to institution); and research funding/trial support from Merck (to institution), Novartis (to institution), Agenus Bio (to institution), Boston Scientific (to institution), Tersera (to institution) and Advanced Accelerator Applications as well as a travel grant for IIT from AstraZeneca. C.E.D. reports research funding from Elicio Therapeutics. A.D.L. reports institutional contracts with Elicio Therapeutics, Bristol Myers Squibb, Exelixis, Arrys Therapeutics, Hutchison Medipharma, Corcept Therapeutics, Conjupro Biotherapeutics and AbbVie for trials where she is the local principal investigator. She serves as Pancreatic Cancer/Neuroendocrine Co-Chair on the Elsevier ClinicalPath Oncology Committee. V.C. has held a consulting or advisory role at Ispen, Gristone Oncology, Westwood Bioscience and Apeiron Biologics; he has been on the speakers’ bureau for Ipsen and Celgene; and he has received research funding from Elicio Therapeutics, Roche and Merck. O.B. reports no relevant disclosures. H.V., L.M.S., A.M.T., J.R.P., E.W., L.K.M., P.C.D., T.K. and C.M.H. are current or former employees of Elicio Therapeutics and, as such, receive salary and benefits, including ownership of stock and stock options, from the company. L.K.M., P.C.D. and C.M.H. have amphiphile vaccine patents pending to Elicio Therapeutics. E.M.O. reports relationships with Rafael Therapeutics (DSMB); Seagen, Boehringer Ingelheim, BioNTech, Ipsen, Merck, Silenseed, Novartis, AstraZeneca, BioSapien, Thetis and Autem, Tempus (consulting/advisory relationship); Agios, Genentech/Roche, Eisai, Zymeworks (spouse: consulting/advisory relationship); and Genentech/Roche, Celgene/Bristol Myers Squibb, BioNTech, AstraZeneca, Arcus, Elicio Therapeutics, Parker Institute, Pertzye and NCI/NIH (research funding).
Gerelateerde artikelen
- Algemene artikelen over immuuntherapie
- Alvleesklierkanker: mRNA vaccin met autogene cevumeran naast atezolizumab en mFolferinox geeft veelbelovende resultaten bij operabele niet uitgezaaide alvleesklierkanker. copy 1
- Baarmoederhalskanker: Immuuntherapie met anti-PD medicijn Cemiplimab plus het vaccin ISA101b gericht op het HPV16 virus of met nivolumab geeft veelbelovende resultaten voor vormen van kanker die HPV gerelateerd zijn
- Blaaskanker: Immuuntherapie met nivolumab en ipilimumab samen geeft hoopgevende resutlaten bij zwaar voorbehandelde gevorderde uitgezaaide blaaskanker copy 1
- Borstkanker: Cryosurgery plus 1x ipilimumab vooraf aan operatie van operabele borstkanker stimuleert immuunreactie en geeft betere therapeutische resultaten
- Buikvliestumoren: immuuntherapie met durvalumab geeft hoopvolle resultaten als behandeling bij gevorderde buikvlieskanker - buikvliestumoren na progressie van ziekte ondanks chemokuren copy 1
- CAR-T cel therapie is een vorm van immuuntherapie die hele goede resultaten geeft. In Jama een overzicht van stand van zaken in de klinische praktijk
- Darmkanker: CYAD-101, een vorm van CAR-T cel immuuntherapie geeft hoopvolle resultaten bij uitgezaaide darmkanker zonder dat graft-versus-host-ziekte ontstaat. copy 1
- Gc-Maf van dr. Yamamoto nu in Nederland beschikbaar
- Hersentumoren: DCVax-L verbetert overall overleving en vermindert kans op recidief bij patiënten met nieuw gediagnosticeerd glioblastoom en recidiverend glioblastoom in vergelijking met beste standaardzorg copy 1
- Histotripsy in combinatie met anti-PD medicijnen nivolumab en ipilimumab wordt onderzocht in fase I studie iFocus in UMC Utrecht
- KRAS gemuteerde tumoren: Kankervaccin ELI-002 2P stimuleerde hoge T-celreacties bij patiënten met voor immuuntherapie ongevoelige KRAS-gemuteerde tumoren en verbeterde de ziekteprogressieve tijd bij patienten met alvleesklierkanker en darmkanker
- Leukemie: Chronische Lymfatische Leukemie: Anti-CD19 CAR T-celtherapie geeft langdurige ziektevrije tijd en duurzame complete remissies bij patiënten met Chronische Lymfatische Leukemie (CLL). copy 1
- Longkanker: Immuuntherapie met Nivolumab + chemotherapie geeft op 3-jaars meting bij patienten met operabele niet-kleincellige longkanker (NSCLC) betere ziektevrije overleving (57 procent vs 43 procent) in vergelijking met alleen chemotherapie
- Lymfklierkanker: Gentherapie voor ernstige oogziekte is opgenomen in de basisverzekering van de ziektekostenverzekering. En geeft hoop voor vergoeding van gentherapie bij vormen van kanker waaronder lymfklierkanker waarvoor gentherapie is goedgekeurd
- Maagkanker: onderhoudstherapie met immuuntherapie met avelumab na chemotherapie geeft verbeterde 2-jaars overleving van 6 maanden in vergelijking met voortzetting van chemotherapie
- Maag en darmkanker: S-1 plus oxaliplatine samen met pembrolizumab geeft hoopvolle resultaten bij patiënten met gevorderde maag / darmkanker met 72 procent objectieve respons
- Melanoom: Mediterraan dieet stimuleert effectiviteit van immuuntherapie met anti-PD medicijnen - checkpointremmers voor patiënten met een melanoom in een gevorderd stadium
- Merkelcel carcinoom: Immuuntherapie met Nivolumab na volledige operatie van Merkelcelcarcinoom verbetert ziektevrije overleving met 11 procent op 2 jaars meting.
- Mesothelioma: Dendritische celtherapie heeft succes bij patienten met mesothelioma - asbestkanker. Erasmus doet oproep dat meer patienten zich aanmelden voor de studie. copy 1
- Mond- en keelkanker: Immuuntherapie met gemoduleerd virus - T-VEC - Talimogene laherparepvec - geeft naast chemo-radiotherapie met cisplatin bij vergevorderde mond- en keelkanker uitstekende resultaten
- Multiple myeloma: Idecabtagene vicleucel (Abecma) geeft als immuuntherapie via CAR-T cellen bij patienten met zwaarvoorbehandelde vergevorderde multiple myeloma uitstekende resultaten
- Neuskanker: Immuuntherapie met Gendicine , een adenovirus P-53 naast chemotherapie geeft uitstekende resultaten bij patienten met neustumoren copy 1
- Newcastle Disease virus vaccin in de behandeling van kankerpatienten geeft extra effectiviteit, blijkt uit verschillende overzichtstudies
- Nierkanker: Nivolumab (Obvio) alleen en samen met ipilimumab (Yervoy) geeft uitstekende resultaten bij gevorderde niercelkanker ook in vergelijking met sunitinib copy 1
- Slokdarmkanker: Nivolumab na neoadjuvante chemoradiotherapie en chirurgie voor patiënten met slokdarmkanker en gastro-oesofageale junctiekanker verdubbelt ziektevrije overleving van 11 maanden naar ruim 22 maanden
- Solide tumoren: Kankerpatienten met solide tumoren met MSI-H = hoge microsatelliet instabiliteit en mismatch reparatie (dMMR) reageren uitstekend op immuuntherapie met pembrolizumab vooraf aan operatie met 65 tot 80 procent complete remissies
- Spijsverteringskanker: Meer bijwerkingen door immuuntherapie met anti-PD medicijn nivolumab bij patienten met spijsverteringskanker geeft superieure resultaten in progressievrije overleving (32 v 8 maanden) in vergelijking met weinig bijwerkingen
- Voeding en suppletie: Dieet - voedingspatroon dat de darmflora beinvloed zoals vezels en probiotica heeft invloed op aanslaan van immuuntherapie met anti-PD medicijnen copy 1
Plaats een reactie ...
Reageer op "KRAS gemuteerde tumoren: Kankervaccin ELI-002 2P stimuleerde hoge T-celreacties bij patiënten met voor immuuntherapie ongevoelige KRAS-gemuteerde tumoren en verbeterde de ziekteprogressieve tijd bij patienten met alvleesklierkanker en darmkanker"